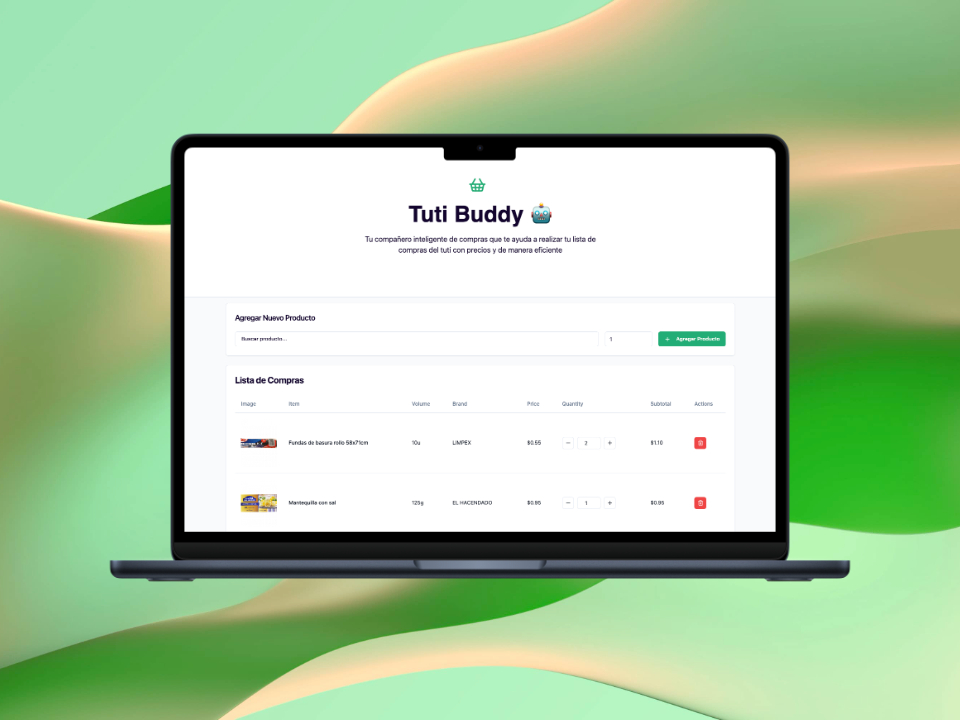
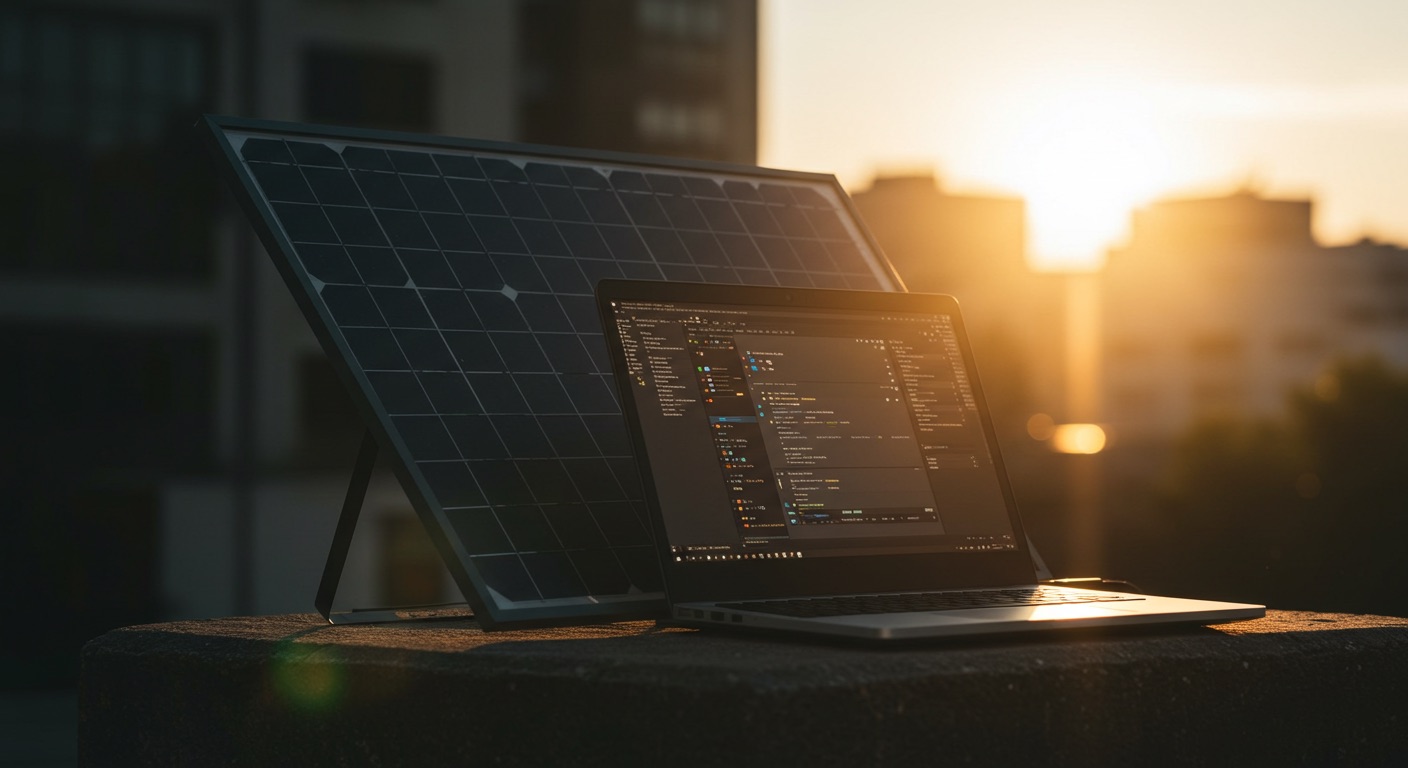
Powering Through - Our Solar Backup Story
A Sustainable Approach for Remote Work during Power Outages using Design Thinking
The extensive blackouts experienced in Ecuador towards the end of 2024 presented a significant challenge for remote work continuity. Facing outages lasting 12 to 14 hours, our team needed a reliable power source to maintain productivity. Using the Design Thinking framework, we developed a solar backup system to power essential equipment and ensure emergency internet access.
1. Empathize Understanding the User Need
News excerpt by Reuters
During the prolonged power cuts, we directly experienced the disruption and uncertainty. Our work, reliant on laptops and internet connectivity, came to a standstill. This firsthand experience helped us empathize deeply with the core problems:
What were we trying to do? We needed a way to keep our essential workstations—specifically four 45W laptops and our WiFi router—powered continuously throughout the blackouts.
Why was this important? Beyond just work productivity, reliable power was crucial for communication and accessing vital information during potential emergencies. The unstable grid threatened our ability to stay connected and informed.
What was currently hard? Our dependence on the national grid made us vulnerable. Existing backup options like generators were often impractical due to fuel scarcity or cost, and smaller battery packs couldn’t sustain multiple devices for extended periods.
From these observations, we understood the core user need: an affordable, self-sufficient power system that could reliably support essential work devices during long outages and recharge independently, coupled with a dependable emergency internet solution.
2. Define Pinpointing the Core Problem
Problem Statement:
How might we design and implement a compact, reliable, and self-sustaining solar backup system to supply uninterrupted power to four laptops and a WiFi router, plus ensure emergency internet access, during extended grid failures?
Objectives:
- Sufficient Energy Output: The system must reliably generate and store enough power to run four 45W laptops and one WiFi router concurrently for the duration of typical blackouts.
- Portability & Scalability: The setup should be relatively compact, easy to assemble, and designed with the potential for future expansion if power needs increase.
- Emergency Connectivity: The solution must include a reliable backup internet source, independent of the local grid, for critical communication.
System Requirements:
Translating these objectives into technical needs, we defined the core components:
- Solar Panel: Minimum 300W capacity to effectively capture solar energy.
- Battery: 100Ah 12V deep-cycle battery for adequate energy storage.
- Power Inverter: 1000W pure sine wave inverter to safely convert DC to AC power for sensitive electronics.
- Solar Charge Controller: 60A MPPT controller to efficiently manage battery charging from the solar panel.
- Emergency Internet: Starlink Gen 3 antenna for grid-independent connectivity.
3. Ideate Brainstorming Solutions
With the problem and requirements clearly defined, we moved into the Ideation phase. We brainstormed various configurations and component combinations. Ideas ranged from using multiple smaller battery packs to exploring different panel types and inverter sizes.
We considered the trade-offs between cost, efficiency, complexity, and portability for each potential setup. Through this process, we converged on the configuration outlined in the System Requirements, as it offered the best balance for our specific needs: sufficient power, relative affordability, ease of assembly, and integration with the Starlink system. The focus was on a practical, achievable solution using readily available components.
-
Solar Panel (Sunpal SP330M-60) to Charge Controller (PowMr POW-M60-PRO)
- Fuse/Breaker: Use a
20A
fuse or circuit breaker (as specified by the panel’s “Maximum Series Fuse” rating). - Wire Gauge (AWG): Use UV-resistant PV wire. Select gauge based on length, targeting <3% voltage drop:
- Up to ~30 ft / 9m:
AWG 14
- Up to ~50 ft / 15m:
AWG 12
- Up to ~80 ft / 24m:
AWG 10
(Ensure chosen wire ampacity meets/exceeds the 20A fuse rating).
- Up to ~30 ft / 9m:
- Fuse/Breaker: Use a
-
Charge Controller (PowMr POW-M60-PRO) to Battery (100Ah 12V)
- Fuse/Breaker: Use an
80A
fuse or circuit breaker (based on 125% of controller’s 60A max output). Place close to the battery/bus bar. - Wire Gauge (AWG): Use
AWG 4
flexible stranded copper wire for short runs (< 5-7 ft / 1.5-2m).
- Fuse/Breaker: Use an
-
Battery (100Ah 12V) to Power Inverter (Renogy 1000W 12V)
- Fuse/Breaker: Use a
150A
fuse or breaker (based on calculation $107A \times 1.25 \approx 134A$, using next standard size). Verify with the Renogy inverter manual for their specific recommendation. Place close to the battery/bus bar. - Wire Gauge (AWG): Keep run extremely short (< 5 ft / 1.5m).
- Absolute minimum for < 3 ft / 1m:
AWG 2
- Recommended for 3-5 ft / 1-1.5m:
AWG 1
orAWG 1/0
(provides safety margin, reduces voltage drop, handles heat better).
- Absolute minimum for < 3 ft / 1m:
- Fuse/Breaker: Use a
-
Controller DC Load Output Terminals (Optional Use)
- Only if using the controller’s specific “Load” terminals for small DC devices.
- Fuse/Breaker: Use an
8A
or10A
fuse/breaker (controller max output is 8A). - Wire Gauge (AWG): Use
AWG 14
for typical short runs (as suggested in controller manual).
-
Starlink Gen 3 Antenna
- This load will be powered by plugging its AC adapter into an outlet connected to the Renogy inverter’s AC output. Its power consumption is well within the inverter’s capacity and is handled by the Battery-to-Inverter wiring calculations (Point 3).
Circuit | Max Current (Rated/Calc) | Recommended Fuse/Breaker | Recommended Wire Gauge (AWG) | Notes |
---|---|---|---|---|
Solar Panel -> Controller | 9.36A (I_sc ) |
20A |
14 (<30ft), 12 (<50ft), 10 (<80ft) |
Use PV Wire. Fuse per panel spec (20A ). |
Controller -> Battery | 60A (Controller Max) | 80A |
4 (< 5-7ft) |
Keep run short. Place fuse near battery/bus bar. |
Battery -> Inverter | ~107A (Calculated) | 150A (Check Manual) |
2 (< 3ft Min), 1 or 1/0 (Rec.) |
Critical: Keep extremely short. Verify fuse in inverter manual. |
Controller DC Load Output | 8A (Controller Max) | 8A or 10A |
14 (Short runs) |
Optional use for small DC loads only. |
4. Prototype: Building the Solution
Transitioning from ideas to a tangible solution, we entered the Prototype phase. We acquired the selected components: the 300W solar panel, 100Ah battery, 1000W inverter, and 60A charge controller.
The initial assembly involved connecting these core components according to standard solar wiring practices. This first build served as our functional prototype – a working model designed to test the feasibility and performance of our chosen concept before full deployment. The Starlink antenna was set up alongside it to simulate the complete emergency backup scenario.
5. Test: Validating and Refining the System
The crucial Test phase involved putting the prototype through realistic scenarios:
Load Testing: We connected the four target laptops (drawing approx. 180W total) and the WiFi router to the system powered solely by the battery and inverter. We monitored battery voltage drop, inverter temperature, and overall system stability under this continuous load to simulate a blackout scenario.
Emergency Internet Verification: We powered the Starlink Gen 3 antenna using the solar backup system during a simulated outage and tested internet speed and stability to ensure it provided reliable connectivity.
Impact: Increased Resilience: Our team’s vulnerability to power outages has been significantly reduced. User Confidence: Knowing we have a reliable backup for both power and internet has eased concerns about future disruptions. Future Enhancements: We are considering adding real-time energy monitoring and potentially expanding the system’s capacity for greater autonomy.
Conclusion
We employed the Design Thinking methodology to address the challenge posed by Ecuador’s blackouts. Grounded in user needs identified via direct experience, we systematically defined objectives, brainstormed solutions, developed a prototype, and conducted rigorous testing. This structured process yielded a reliable solar backup system. Importantly, the project concurrently developed our practical skills in applying a user-centered framework, enhancing our capacity for resilient problem-solving.
📬 Your friendly dose of UX, IT, and DIY tips!
I deliver actionable tips for enhancing productivity, practical life advice, and top-notch insights gathered from various sources, right to your email inbox.